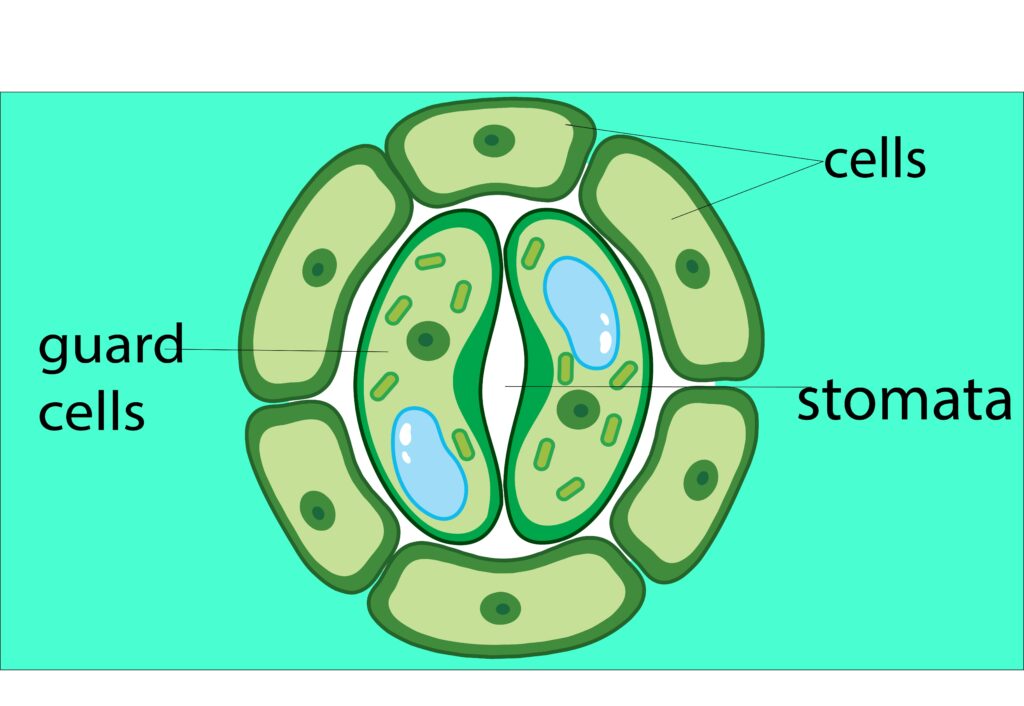
Introduction:
Stomata, those microscopic pores on the surface of leaves, play an indispensable role in the life of plants. These gatekeepers of plant vitality are responsible for the exchange of gases, enabling the vital processes of photosynthesis and respiration. In this blog, we’ll delve into the fascinating mechanism of opening and closing stomata exploring the relation between plant survival and environmental adaptation.
The Significance of Stomatal Regulation
Stomata, primarily found on leaves, stems, and other aerial plant parts, regulate the influx of carbon dioxide and the outflow of oxygen during photosynthesis, ensuring efficient energy production. They also manage water loss through transpiration, a crucial process for maintaining the plant’s water balance.
Anatomy of Stomata
The structure of a stoma consists of two specialized guard cells that flank a central pore. These guard cells are equipped with chloroplasts and are connected by subsidiary cells, forming a dynamic ensemble responsible for stomatal movement. Guard cells are bean-shaped and are typically oriented with their long axis perpendicular to the leaf surface. This orientation allows the guard cells to regulate the opening and closing of the stoma effectively. Accompanying the guard cells are subsidiary cells, which support their function. The size of the stomatal pore is a critical factor in determining the rate of gas exchange and water loss. When guard cells swell and bow outward, the pore opens, facilitating the entry of carbon dioxide for photosynthesis and the exit of oxygen and water vapor. Conversely, when the guard cells lose turgidity, the pore closes to prevent excessive water loss.
Mechanism of Opening and closing of stomata
The opening and closing of the stomata is driven by various factors, with light being the primary trigger. Photoreceptors in guard cells perceive light, causing the accumulation of potassium ions within the guard cells. This osmotic influx of ions increases the turgor pressure, leading the guard cells to swell and bow outward, thus creating a larger pore for gas exchange.
Role of Blue Light in Stomatal Response
Blue light has a particularly influential role in stomatal opening. Phototropins, the blue light receptors, activate proton pumps in the guard cells’ plasma membrane. This proton influx results in pH reduction within the cell wall, facilitating cell expansion and stomatal pore formation.
Imagine blue light as a plant’s wake-up call. Blue light, with its shorter wavelength, is highly energetic and can trigger photoreceptors known as phototropin present in the guard cells of stomata. This phototropin, like nature’s light sensors, absorbs blue light and sets off a cascade of events that leads to a stomatal opening.
Upon absorbing blue light, phototropin activates proton pumps in the plasma membrane of guard cells. These pumps transport protons (H+) out of the guard cells, creating a localized proton-rich environment. This proton gradient is a crucial part of the stomatal response mechanism.
Acidification of Cell Wall
The transport of protons by proton pumps leads to the acidification of the cell wall surrounding the guard cells. This drop in pH activates enzymes that modify the cell wall’s components, making it more flexible and elastic.
Potassium Ion Movement
As the cell wall becomes more flexible, it allows the guard cells to take in potassium ions (K+). This influx of potassium ions increases the osmotic concentration within the guard cells, resulting in water uptake. As a result, the guard cells swell and bend outward, creating an opening known as the stomatal pore.
CO2 Uptake and Transpiration
The open stomatal pore not only allows for the influx of carbon dioxide (CO2), a vital ingredient for photosynthesis but also facilitates the escape of oxygen produced during this process. Additionally, the opening of stomata leads to water loss through transpiration, a process that aids in maintaining the plant’s water balance.
Blue Light Intensity and Stomatal Behavior
The intensity of blue light plays a crucial role in determining the extent of stomatal opening. Higher intensities of blue light can lead to wider stomatal pores, allowing for increased gas exchange and photosynthesis. However, in excessively intense light, stomata might begin to close to prevent excessive water loss.
Environmental Factors and Stomatal Closure
Stomatal closure is vital to prevent excessive water loss, especially in challenging environmental conditions. Factors like high temperatures, low humidity, and water scarcity trigger the closure of stomata. Abscisic acid (ABA), a plant hormone, plays a pivotal role in this process by promoting the efflux of potassium ions and decreasing turgor pressure.
Role of High Temperatures in Closure
One of the primary triggers for stomatal closure is high temperature. When temperatures soar, plants risk excessive water loss through transpiration. In response, they close their stomata to conserve water and prevent dehydration.
Low Humidity: The Drying Effect
Low humidity is another environmental factor that prompts stomatal closure. When the air is dry, the gradient between the moisture inside the leaf and the surrounding air increases, accelerating water loss. Stomatal closure under such conditions reduces this risk.
Water Scarcity: A Survival Strategy
Plants facing water scarcity employ a preemptive strategy by closing their stomata. This prevents water loss and helps maintain turgidity, which is essential for structural support and effective nutrient transport within the plant.
Windy Conditions and Stomatal Closure
Strong winds accelerate transpiration by removing the thin layer of moist air that surrounds the stomatal pores. In response, plants close their stomata to minimize water loss, preserving precious water resources.
Darkness and Stomatal Behavior
During the night, when photosynthesis is not occurring, plants tend to close their stomata to prevent unnecessary water loss. This closure conserves water and helps maintain favorable internal conditions.
The Role of ABA: An Adaptive Hormone
Abscisic acid (ABA), a plant hormone, plays a pivotal role in stomatal closure. When environmental cues signal the need for closure, the plant synthesizes ABA which triggers the efflux of potassium ions from guard cells. This leads to water loss and subsequent stomatal closure..
ABA’s Role in Stomatal Opening and closing stomata
Under certain conditions, ABA can promote stomatal opening. For instance, during low light or shaded environments, ABA can act in conjunction with other hormones like auxins to induce stomatal opening. This allows plants to optimize carbon dioxide uptake, even in less optimal lighting conditions.
ABA’s Signaling Pathway for Closure
The mechanism behind ABA-induced stomatal closure is intricate. When ABA binds to specific receptors in guard cells—the cells that flank the stomatal pore—it triggers a signaling cascade. This cascade leads to the efflux of potassium ions from guard cells, which reduces turgor pressure and causes water to exit the cells. The loss of turgor pressure prompts the guard cells to close, effectively closing the stomatal pore.
Stomatal Sensing: Osmotic Regulation
Guard cells are sensitive to changes in osmotic pressure. When water availability decreases, the osmotic concentration inside guard cells increases, causing water to leave the cells. This loss of turgor pressure prompts stomatal closure. In response to environmental cues like high temperatures or water scarcity, guard cells employ osmotic regulation to effect stomatal closure. Guard cells actively transport potassium ions out of their cytoplasm, reducing the osmotic potential inside the cells. This prompts water to exit the guard cells, causing them to lose turgor pressure and leading to stomatal closure.
Plants that exhibit efficient osmotic sensing and regulation are better equipped to withstand drought conditions. By closing their stomata in response to decreasing water availability, these plants conserve water and maintain their physiological functions. The ability of guard cells to sense osmotic changes involves the coordination of various genes and metabolic pathways. The expression of genes related to ion transport and osmolyte accumulation contributes to stomatal response.
Guard Cell Sensing: A Water-Saving Strategy
Guard cells sense changes in humidity through osmoregulation. When humidity drops, guard cells lose water, causing them to become flaccid and leading to stomatal closure. This mechanism prevents water loss and ensures the plant’s survival during arid conditions.
The Dance of Stomata: Circadian Rhythms
The stomatal activity follows a circadian rhythm. During the day, stomata are open to facilitate photosynthesis, while at night, they are close to minimize water loss. This synchronized pattern helps plants optimize their energy usage and water conservation.
Stomata and Photosynthesis: A Synchronized Effort
Stomatal behavior is intricately linked with photosynthesis, the process by which plants convert light energy into chemical energy. Circadian rhythms ensure that stomata open during daylight hours when photosynthesis is most active, facilitating the exchange of carbon dioxide and oxygen.
Light-Dark Cycles and Stomatal Opening
As day breaks and light floods the landscape, plants sense this change through specialized receptors. The circadian clock synchronizes stomatal opening with the availability of light, ensuring efficient carbon dioxide uptake for photosynthesis.
The Night Shift: Stomatal Closure
When night falls, plants transition to a phase of stomatal closure. Circadian rhythms dictate the timing of this closure, preventing unnecessary water loss during the dark hours when photosynthesis is inactive.
Temperature and Circadian Rhythms
Temperature fluctuations also influence stomatal behavior. Circadian rhythms interact with temperature cues, regulating the degree of stomatal opening to prevent water loss during hot periods and conserve water during cooler periods.
Human Influence on Stomatal Behavior
Human activities, such as deforestation and pollution, can disrupt the delicate balance of stomatal function. Increased carbon dioxide levels can lead to reduced stomatal density, affecting the plant’s ability to effectively regulate gas exchange and water loss.
Engineering Stomatal Behavior for Crop Improvement
Scientists are exploring genetic modifications to enhance crop resilience by manipulating stomatal behavior. By developing plants with improved water-use efficiency and enhanced CO2 uptake, researchers aim to address food security challenges in a changing climate.
Conclusion: Nature’s Responsive Pores
In essence, stomata are remarkable structures that exemplify nature’s ingenuity. Their ability to respond to environmental cues in real-time showcases the intricate balance between survival and adaptation. Understanding the mechanism of stomatal opening and closing not only deepens our appreciation for the natural world but also offers insights into sustainable agricultural practices and environmental stewardship.
FAQs (Frequently Asked Questions)
Can animals have stomata too?
No, stomata are unique to plants and are absent.
How does humidity affect stomatal behavior?
High humidity promotes stomatal opening, while low moisture triggers stomatal closure.
Are all guard cells the same shape?
No, guard cells can vary in shape and size depending on the plant species.
Why is ABA necessary for stomatal closure?
ABA promotes the efflux of potassium ions, leading to water loss from guard cells and subsequent stomatal closure.
How are circadian rhythms linked to stomatal activity?
Circadian rhythms regulate the timing of stomatal opening and closure, optimizing gas exchange and water conservation.
No Responses